Knowing how to improve your aerobic endurance through training can be confusing. Responses to exercise are very individualized, and endurance adaptations largely occur at the biochemical level, which makes it challenging to decide what approach to take. In this article, we’ll discuss some of the changes that occur on the cellular level and how to leverage them for your performance gains.
Understanding the science and physiology behind endurance training will help you make the right training decisions.
VACUUM ANALOGY FOR LACTATE SHUTTLE
In our books, Training for the New Alpinism and Training for the Uphill Athlete, and an article on why we are forced to slow down, the concept of a lactate “vacuum” is referenced, which is scientifically known as the intracellular lactate shuttle.
The lactate shuttle is a widely-used theory in which transporter proteins move lactate, accumulated during anaerobic exercise, from muscle fibers without mitochondria into fibers with a high density of mitochondria.
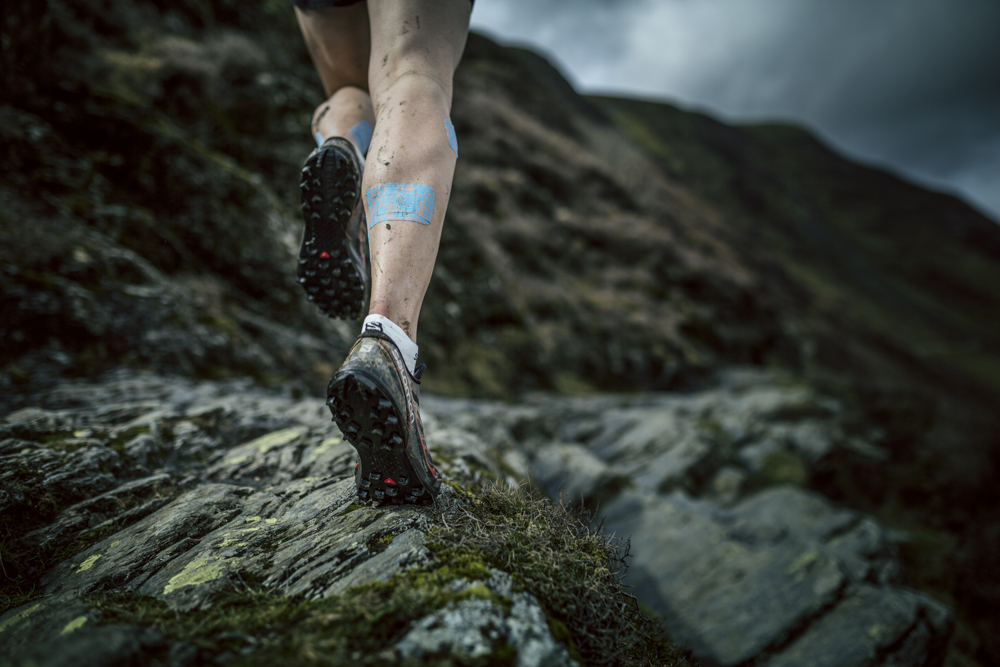
By understanding the science of endurance training, you can achieve sustainable advances in your training and performance.
Lactate that builds up during exercise can be reused for energy in two ways.
- Taken to the liver and converted back into glucose, or
- Oxidized in the mitochondria and cycled back into molecules contributing to ATP production.
Suppose lactate is not cleared out and reused for energy. In that case, it will continue to build up as exercise intensity increases and cause a change in blood pH that affects muscle function, and eventually, intensity must decrease. Converting lactate to glucose in the liver is a slow process and cannot be relied on to provide energy during exercise, so our focus is the lactate shuttle.
The “vacuum” analogy describes the lactate shuttle in two ways.
- Transporter proteins move the lactate accumulated during intense exercise into muscle fibers with mitochondria — the transport rate can be increased by training at or above your lactate threshold or other high-intensity workouts.
- Mitochondria in your muscles receive the lactate and oxidize it into molecules to create energy to sustain exercise. As all good students of Uphill Athlete know, mitochondrial density is increased through aerobic base training.
In almost all cases, the intracellular lactate shuttle in our working muscles moves lactate from type IIA & IIX (fast twitch) muscle fibers, which have far fewer and far smaller mitochondria, into type I (slow twitch) fibers, which posses far more and larger mitochondria.
Therefore, increasing the mitochondrial density in your type I fibers through aerobic base training expands the size of your vacuum.
If there is no site to use the lactate, then we can also train the rate of shuttling. For this, the primary goal of all endurance athletes should be to expand the size of their “vacuum”.
While some aspects of endurance physiology, such as VO2max, distribution of muscle fiber types, and biomechanics are relatively fixed by genetics, increasing the number of mitochondria in your muscle cells is very trainable. Exercising at low intensities (heart rate zones 1 & 2) are generally considered optimal for stimulating mitochondrial biogenesis in the body.
A solid understanding of the physiology behind endurance training helps to trust that you are doing the right training to develop your energy pathways and lactate shuttle. Improvements are not always linear, but by understanding the science and suggestions below, you can develop sustainable advancements in your training and performance.
WHAT IS ENDURANCE TRAINING?
Improving endurance through training is based mainly on improving the energy pathways that rely on oxygen to help fuel them. The best endurance workouts will focus on one pathway at a time to maximize the improvement of that system.
Our body relies on three main energy pathways (two anaerobic and one aerobic) in life and exercise. The goal of each is to create ATP (adenosine triphosphate), our primary energy source.
The two anaerobic pathways are phosphocreatine (PCr) and anaerobic glycolysis, which quickly create energy but are depleted in seconds or minutes. Some sprinters will rely entirely on these energy systems, but working at the paces required to use these pathways is unsustainable for endurance athletes. The build-up of glycolytic byproducts (including lactate) is so intense that they cannot be cleared before they affect muscle function.
Then we have aerobic glycolysis, the primary engine of aerobic endurance activities. The “vacuum” for lactate and other byproducts firmly resides within this system. Compared to the PCr and anaerobic glycolytic systems, aerobic glycolysis produces significantly more ATP but much slower. Aerobic glycolysis is based primarily on converting macronutrients, mostly dietary, into molecules that can enter the electron transport chain inside our mitochondria, where ATP is produced to fuel physical activity.
The electron transport chain is located in mitochondria, which are found in muscle fibers with large endurance and oxidative capacities. In mitochondria, we see lactate uptake, where it is oxidized and turned back into energy.
This occurs via conversion to another molecule, pyruvate, as facilitated by the enzyme lactate dehydrogenase (LDH). Once in pyruvate form, a key molecule in glycolysis, it can be further reduced to another molecule, acetyl COA, and enter the citric acid cycle (TCA). The citric acid cycle is a biochemical process that produces many energy production precursors, sometimes called the Krebs Cycle. The TCA will now produce the molecules necessary for creating ATP in the electron transport chain.
The oxidation of lactate and subsequent conversion to an energy-producing intermediary (pyruvate) occurs in the mitochondria. Therefore, building up the number of mitochondria in your body will increase your ability to create aerobic energy and utilize the byproducts of anaerobic energy. This process’s complexity also helps explain why producing energy through aerobic glycolysis is not a quick process, compared to anaerobic glycolysis.
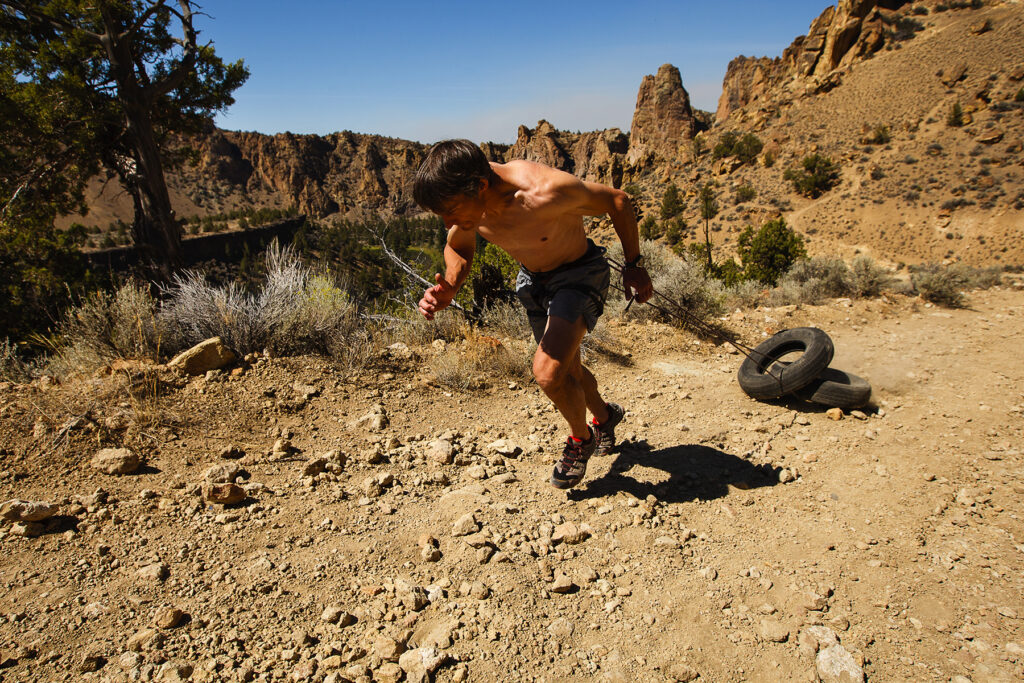
Muscular endurance training, done here by Steve House while training for Nanga Parbat in 2005, will not develop aerobic glycolysis development.
These energy pathways do not exist in isolation, nor do we “switch” between them, as with most physiological processes, there are overlaps and systemic redundancies. While there are two thresholds, aerobic and anaerobic, which dictate whether glycolytic byproducts (primarily lactate) will begin to accumulate to levels that require a decrease in exercise intensity, the aerobic system does not completely shut off when you start to rely on anaerobic energy sources.
This means that we are capable of accelerating and decelerating as needed. If our energy systems didn’t overlap, we couldn’t perform sudden bouts of hard work. For example, when hiking over a mountain pass, you rely primarily on aerobic glycolysis if you’re relaxed and breathing comfortably. But suppose the weather starts to roll in, and you need to move quickly on the steeper sections of your climb. In that case, you might be producing energy anaerobically if you are not well-trained. Depending on your level of training, you start producing energy aerobically again when the terrain is more manageable.
To summarize, as intensity increases, aerobic glycolysis will start to hand off the responsibility of keeping you moving to anaerobic glycolysis because oxygen demands are higher. As intensity decreases and oxygen demands are lower, the responsibility returns to aerobic glycolysis. The smooth transition of energy production between each system prevents any lag or gap in ATP creation so that we can rev our engines up or down as needed.
Based on this idea of moving quickly on a mountain, it’s important to remember that lactate accumulation in your body will fluctuate depending on what system you’re primarily using for energy. However, these fluctuations will matter a lot more in an aerobically untrained individual because they lack the vacuum components to use their lactate.
In an aerobically trained individual, not only can they shuttle their lactate off to make more energy, but the intensity needed to accumulate blood lactate is much higher. This is because they have an aerobic base capable of producing energy aerobically at more increased efforts than the untrained individual. They can work harder for longer without relying on anaerobic glycolysis.
CAN ATP STORES BE TRAINED?
Given the importance of ATP, it’s a natural question to ask if we can increase the amount of ATP in our energy systems or store more of the molecule to skip aerobic glycolysis. The answer is no, primarily because it is a relatively unstable molecule without a biochemical storage location inside the body.
Since our bodies use ATP for almost all non-exercise functions, it is tightly regulated. A secondary reason is weight. Regarding molecular weight, ATP is phenomenally heavy, and mass storage would make humans immobile. What does this mean for you as an athlete?
As with all things endurance, all roads lead towards increasing your aerobic capacity so that you can more effectively leverage aerobic glycolysis and the lactate shuttle to produce more energy at a lower cost to your muscles and respiratory system.
TYPES OF ENDURANCE TRAINING
There are several ways to train your ability to clear and use the lactate produced by anaerobic glycolysis. The below cardiovascular endurance exercises will create a platform for continued performance in your chosen sport.
- Build your aerobic base: Increasing your aerobic capacity leads to mitochondrial density, which will build up the lactate utilization infrastructure in your cells. It gives the shuttled lactate somewhere to go for utilization.
- Train your lactate threshold (LT): By training near or at your lactate threshold (the maximum intensity you can continue to clear lactate), you can build your ability to shuttle or convert lactate back into glucose effectively. Building your aerobic base will increase your mitochondrial density, and training your lactate threshold will boost effectiveness.
- Fartlek or tempo interval training: Fartlek and tempo interval training involve increasing your exercise intensity above your aerobic or anaerobic thresholds, then bringing the intensity back down to a leisurely aerobic pace to clear out the built-up lactate. Fartlek training tends to be over a longer distance, and therefore your high-intensity periods are less likely to be maximal efforts. Still, you want to exceed your aerobic capacity to introduce strain into the system. The same is true in tempo intervals, but you might work at a higher intensity depending on your training goals and sport. In both cases, intervals have been shown to cause less accumulated blood lactate post-exercise and, therefore, less strain on the body.
While these are established methods of training your body to handle better and utilize lactate, there has been a lot of discussion over the practical application of these training methods. The endurance training community has a long-standing debate over volume versus intensity, which could also be stated as quantity versus quality.
Individuals who prefer or find more success in weeks of high-volume activity (for example, the 200-mile weeks that some professional marathon runners will complete leading up to competition) rely on the massive contributions that such training makes to their muscular endurance and aerobic base via mitochondrial biogenesis.
People who respond better to training at the lactate threshold, and other more intense work, build on a natural ability to clear lactate. In both cases, as described in this article, one cannot live without the other. Practically, it makes more sense to focus on building a solid aerobic base while considering that intensity will help sharpen your overall race intensity performance.
HOW TO IMPROVE MUSCULAR ENDURANCE?
While the primary driver behind performance in endurance activities will be improved energy pathways, muscular endurance also plays an important role. Put simply, if you have a powerful engine, but a poorly built frame, you won’t be able to make the most effective use of your energy-creation abilities.
Types of muscular endurance are based on the types of muscle fibers recruited for a task. Humans have three types of muscle fibers: I (slow twitch muscle fibers) and IIA + IIX (fast twitch muscle fibers).
Type I fibers have a high level of endurance and aerobic capacity and fire with a lower force than the other types but can do so many more times. Postural muscles, such as your lumbar, abdominals, and calf muscles, are mostly type I because they need to be highly fatigue resistant to keep you standing up. Muscles like the biceps and quadriceps will have higher percentages of type IIA and IIX. Still, it’s important to remember that each muscle has a mixture of these fibers, and the percentages of fiber types in your muscles are based on genetics and training.
Type I fibers are the mitochondrially dense fibers and, therefore, the type we want to recruit the most for endurance activities. Fast twitch fibers will have few or no mitochondria, so energy pathway development is less important than the Type I fibers.
The focus is to recruit our Type I fibers for repeated bouts of relatively low-intensity exercise to improve muscular endurance.
A QUICK NOTE ON ENDURANCE VS. STRENGTH TRAINING
Strength is built on the repeated maximal or near-maximal recruitment of a high number of muscle fibers. The energy pathways used to recruit muscle fibers in quick bursts, such as a heavy deadlift, are much less of a factor than endurance sports because strength building occurs in very short sets lasting around 20-30 seconds. Strength training will help develop muscular endurance but will not develop aerobic glycolysis development.
CONCLUSION
In conclusion, understanding the science behind endurance training can be challenging due to the individualized responses to exercise and biochemical changes at the cellular level. This article discussed the concept of the intracellular lactate shuttle, which moves lactate from muscle fibers without mitochondria into fibers with high mitochondrial density. Increasing mitochondrial density through aerobic base training can expand the lactate “vacuum” size and improve endurance.
Endurance training primarily focuses on improving the energy pathways that rely on oxygen. Our body has three main energy pathways (two anaerobic and one aerobic), with the aerobic glycolysis system being the primary engine of aerobic endurance activities. This process produces significantly more ATP but at a slower rate.
Improving your endurance through training is possible by improving the aerobic glycolysis system and building up the number of mitochondria in your body. A solid understanding of the physiology behind endurance training will help you develop sustainable increases in your performance.
The Science and Physiology of Endurance Training
Table of Contents